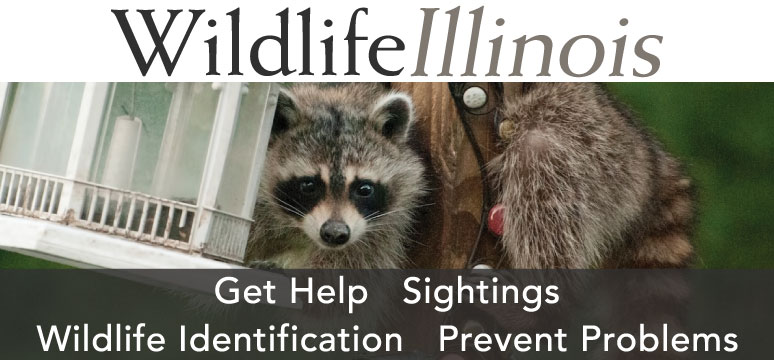
The increase in Cooper’s hawk populations in recent years can be at least partially attributed this predator finding easy food at backyard feeders.
The increase in Cooper’s hawk populations in recent years can be at least partially attributed this predator finding easy food at backyard feeders.
Photos by Travis Wilcoxen
A great-horned owl was admitted to the Illinois Raptor Center (IRC; a wildlife rehabilitation facility in Decatur) in September 2018 with several sores in its mouth. It was starving, covered with parasitic hawk flies, and was severely anemic. This bird had no broken bones and no other signs of trauma. With no other tell-tale indicators of the specific cause of this illness, no additional diagnosis was made and the bird died one day later. There are very few quick tests available to identify wildlife diseases, and unfortunately, that means many animals die without a diagnosis that could have provided important information about what diseases might be spreading. In 2022, the blood sample from that owl, which had been stored frozen, was retrieved and included in a broad survey for rabbit fever (tularemia), along with hundreds of other samples. It was positive. Four years after that sample was collected, we started to understand what symptoms we might expect in birds of prey with rabbit fever.
Disease is an important factor that impacts wildlife populations at lethal and sub-lethal levels. Disease-causing agents, such as bacteria, viruses, fungi, prions and parasitic protists, have always been present in animal populations. In addition to raising concerns about the health of wildlife populations, as disease spreads in wild animals, it greatly increases the risk of the disease infecting humans if it is already capable of doing so, or creating new situations where the disease-causing agent evolves to bypass the human immune system, and a new zoonotic disease emerges. These zoonotic diseases – diseases that move from non-human animals into humans – account for more than 60 percent of all emerging diseases worldwide (WHO, 2023).
In 2006, Dr. Roger Mahr, President of the American Veterinary Medical Association (AVMA) called for a commission that would guide the merger of concepts, strategies, and data in human and veterinary medicine, and in 2006, the One Health Commission was formed in 2009. Since that time, nearly 1,000 medical professionals, veterinarians, disease ecologists, and other scientists have endorsed, or contributed to, the work of the commission and participation is now global.
Organizations like the OneHealth commission, government agencies, and disease ecologists, like myself, have increased efforts in monitoring disease moving among livestock, companion animals, wildlife, and humans, with an intentional focus on gaining a better understanding of shared diseases among all animals. This is critical to improving human health, livestock and companion animal health, and wildlife health.
Not all diseases in wildlife pose a great risk of becoming zoonotic. There are several factors that contribute to a full assessment of risk, but in general, diseases are more likely to spillover from non-human mammals to humans, followed by spillover of diseases from birds to humans, with disease spillover from other animals to humans being very rare. Those wildlife diseases that are not currently human diseases (i.e., not zoonotic) are still important players in population dynamics of wildlife and are of critical importance when considering wildlife management plans, population health and conservation strategies.
There are many disease surveillance techniques used in monitoring wildlife populations, including necropsy of dead animals to examine signs of disease beyond the outward appearance, and polymerase chain reaction (PCR) testing of body fluids to detect genetic material from the disease-causing agent itself. However, surveillance using necropsy or PCR provides data only on currently infected and currently not-infected individuals. Another technique, and one used extensively in our lab, is enzyme-linked immunosorbent assay (ELISA) to detect antibodies generated by the wildlife against the specific disease-causing agent. Elevation of some antibody types are indicators of a recent infection while others are indicators of a history of exposure well beyond the clearance of the disease from the system. While we work with fish, amphibians, reptiles, and birds in our lab at Millikin University, our primary focus in disease surveillance is in birds, and specifically small birds, such as songbirds, and birds of prey or raptors.
Among many diseases studied at the Wilcoxen Lab at Millikin University, one that we have explored the most is Mycoplasma conjunctivitis (bird pink eye) in songbirds that use feeders and in birds of prey. We found that birds physically affected by pink eye are much more common at sites with human-provided bird feeders compared to birds captured at natural areas where no feeders are present (Wilcoxen et al. 2015). The disease disproportionately affects finches and can lead to significant declines in the number of finches at sites where outbreaks occur, but it does not appear to cause similar declines in non-finch species, despite antibody evidence that they have contracted the bacteria at some point in time (Vana et al. 2018). Those same small birds are common prey items for larger birds of prey. One could imagine that a predatory bird consuming a bird would leave them susceptible to the diseases of the prey, but the general principle of predators taking the sick and the weak seems to be a good strategy here. We surveyed birds of prey admitted to the IRC for rehabilitation, and found that none of them had pink eye, but 41 percent of them had antibodies to the bacteria that causes pink eye, with a percentage much higher in birds that commonly eat birds, such as Cooper’s hawks (72.3 percent, Wrobel et al. 2015). Our surveillance allowed us to determine that although pink eye at feeders is a problem for the finches, most birds develop antibodies and do not become ill (Vana et al. 2018). Further, it does not seem likely that outbreaks of pink eye in feeder birds are causing problematic disease transmission to the predators of those birds (Wrobel et al. 2015).
Surveillance of diseases in populations of wild birds can also provide important information about zoonotic disease. Samples from migratory birds provide the best predictors of variation in the annual influenza viruses that move through the human population, as those newly mutated strains are carried over thousands of miles in the respiratory tract and feces of migratory birds, dropped into areas developed by humans, then spread within the human population (Webster et al. 2006; Krauss and Webster 2010). While influenza has not been the focus of our research, one virus that we have studied in wild birds is West Nile Virus.
West Nile Virus (WNV) was first documented in the United States in 1999 in New York and has spread westward and southward since (Hayes et al. 2005). WNV is transmitted by mosquitoes, and there are active mosquito-WNV surveillance practices in nearly every major municipality within the WNV range aimed at alerting the public and health care providers of an increase in the viral threat. However, the mosquitoes are not where the virus resides and changes; instead, they are temporary carriers during a short window of a few months in the summer and early fall. Birds are the primary reservoir host for WNV, with some birds dying from it, and some birds clearing the infection. Most birds tolerate the virus and live with low-level infection. Our work has found that songbirds surviving a WNV infection tend to be higher-quality birds with a high likelihood of surviving with low-grade infections (Plants and Wilcoxen 2016). Bird to mosquito to human transfer is the primary path to WNV infection in humans. Blood samples collected from birds in the early spring and early summer could provide an even earlier indicator of the risk of WNV transmission when mosquito populations reach a critical mass to transmit. Indeed, we found the prevalence of West Nile Virus antibodies in birds to decrease significantly during the winter, followed by a steady rise in the spring and spike in summer that persists through the fall (Pulver et al. 2021), but that it reaches a high prevalence in birds well before most municipal mosquito surveys produce a WNV-positive mosquito.
Another zoonotic disease of interest due to its transmissibility to humans is tularemia, or rabbit fever. This is caused by a bacterium, Francisella tularensis, and is most frequently harbored by small mammals, such as rabbits. Of course, this puts the pathogen in direct contact with anything hunting these small mammals – including human hunters and birds of prey. Surveying hunted game or human hunters themselves for exposure or infection can create logistical challenges, but with access to hundreds of blood samples from birds of prey annually, we have been able to get a sense of how common this disease is in their prey, as direct contact with an infected animal is the primary mode of transmission. In a survey of birds of prey admitted to the IRC from 2018 to 2022, 14.1 percent of the birds had antibody evidence of a tularemia infection, while expressing no signs of illness associated with that disease (Rockwell et al. unpublished). A similar, annual survey of these birds could provide insight into the probability that hunters will encounter an infected animal and increase awareness of the disease, encouraging additional precaution when handling the carcass. Visit the Wildlife Illinois website to learn more about tularemia.
Whether it be for the sake of conserving populations of wild animals or understanding the risk of spillover of disease from wild animals into humans, surveillance of the prevalence of diseases in populations of wild birds can be very useful. Hopefully with increased awareness of the inter-connected nature of human health, wildlife health, and livestock and companion animal health, informed by disease data from these populations, we will be able to establish better practices that improve the health of all.
Hayes, E.B., et al. (2005) Emerging Infectious Diseases 11:1167-1173.
Krauss, S. and R.G. Webster (2010). Avian Diseases 54:394-398.
Plants, S. and T.E. Wilcoxen (2016). BIOS 87:131-137.
Pulver, O., T.E. Wilcoxen, J.T. Nuzzo, and J. Seitz (2021) Current Topics in Virology 18:47-63.
Vana, E.R., E.R. Wrobel, and T.E. Wilcoxen (2018). Avian Biology Research 11:7-11.
Webster, R.G., M. Peirls, H. Chen, and Y. Guan (2006). Biodiversity 7:51-55.
Wilcoxen, T.E. et al. (2015) Conservation Physiology 3: cov058
World Health Organization (2023) https://www.emro.who.int/about-who/rc61/zoonotic-diseases.html
Wrobel, E.R., T.E. Wilcoxen, J.T. Nuzzo, and J. Seitz (2015). Journal of Raptor Research 50: 289-294.
Dr. Travis Wilcoxen is a Professor of Biology at Millikin University in Decatur. He teaches courses in immunology, anatomy, and physiology, as well as ecology, evolution, and biostatistics. As a physiological ecologist, his research includes immune function, physiology and disease ecology of birds, amphibians, fish, and reptiles. He graduated with a Bachelor’s Degree in Biology from Eureka College in 2005, and a Ph.D. in Vertebrate Zoology from the University of Memphis in 2010.
Submit a question for the author